Numerical models are some of the principal tools for understanding the cycling of geochemical and biogeochemical tracers in the ocean, with the latter also being important components of the Earth System Models used to project future climate change. However, in order to use these models they must first be integrated to a seasonally-repeating equilibrium with minimal drift, a computationally expensive calculation that can take months on supercomputers given the long turnover timescale – many thousands of years – of the ocean. This “spin-up” problem has long been a major bottleneck in marine geochemical and biogeochemical modelling.
In a study published last year in J. Adv. Model. Earth Sys (2023, see reference below), a new algorithm was shown to speed-up by a factor of between 10-25 the spin-up of a wide range of geochemical tracers, such as radiocarbon, protactinium/thorium and zinc. It can be applied to any model in a “black box” manner.
Now, a follow up study published recently in Sci. Adv. (2024, see reference below) extends the previous results to complex marine biogeochemical models such as those used in the Coupled Model Intercomparison Project (CMIP) that underpin IPCC reports on climate change. The algorithm can accelerate the spin-up of seasonally-forced models by over an order of magnitude, and by a factor of 5 when driven with interannually forcing as is typical in CMIP simulations.
The ability to efficiently spin-up geochemical and biogeochemical models should enable their more effective use, for example making it feasible to calibrate models against observations and performing simulations at resolutions higher than has been previously possible.
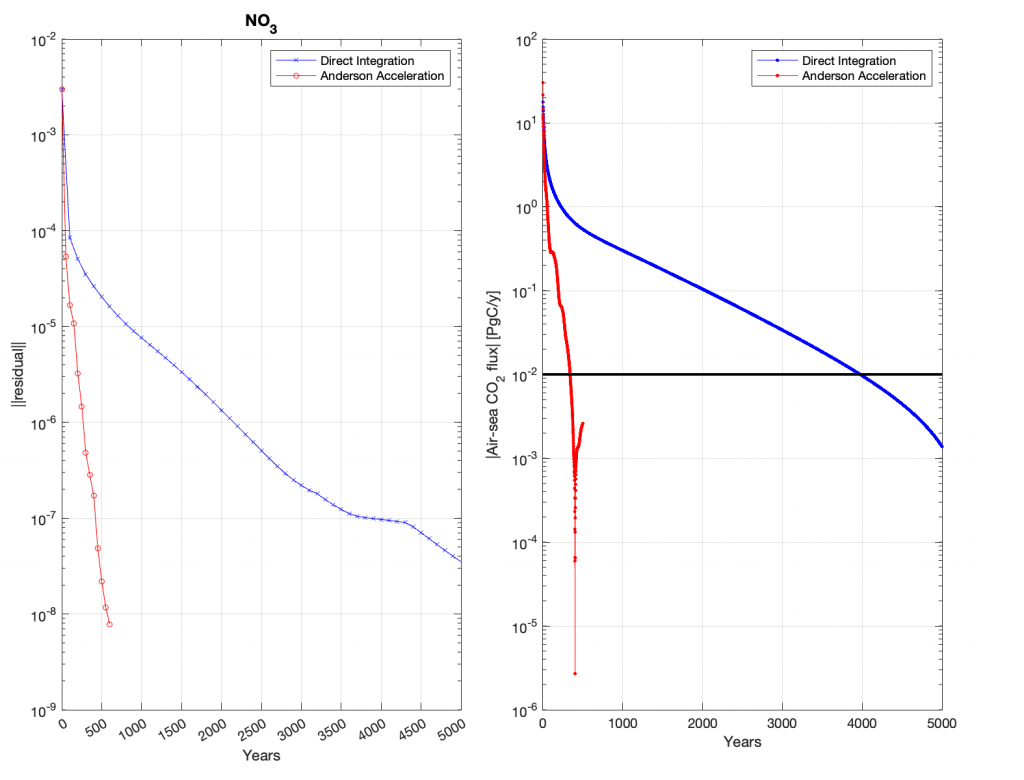
Caption: Spin-up to equilibrium of the PISCES marine biogeochemical model. PISCES is coupled to the NEMO ocean circulation model and has 24 prognostic tracers. Left: Drift in dissolved inorganic nitrate concentration (mean squared difference at all grid points between consecutive years) as a function of time. Right: Globally-integrated air-sea flux of CO2 as a function of time. The solid horizontal line is the criterion for convergence established by the Ocean Model Intercomparison Project (OMIP). The blue lines are the conventional direct integration solution and the red lines the accelerated solution using the new algorithm.
This is a joint highlight with the GEOTRACES program.
Reference:
Khatiwala, S. (2024). Efficient spin-up of Earth System Models using sequence acceleration. Science Advances, 10. Access the paper: 10.1126/sciadv.adn2839
Khatiwala, S. (2023). Fast Spin‐Up of Geochemical Tracers in Ocean Circulation and Climate Models. Journal of Advances in Modeling Earth Systems, 15. Access the paper: 10.1029/2022ms003447