With an increasingly wide variety of technology and innovations, from buoys to satellites, we now understand the open ocea n better than ever. Yet, existing technologies cannot cost-effectively provide accurate, up-to-date data on coastal and shelf ocean environments, especially beneath the surface. These dynamic regions impact billions of people in profound and varied ways.
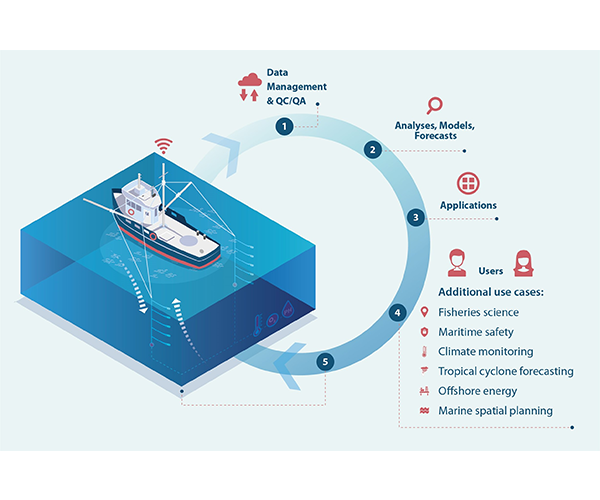
Figure caption: Alongside other major global ocean observing technologies and networks, the Fishing Vessel Ocean Observing Network is built around the concept of “fishing for data” to collect high-quality ocean data such as temperature and salinity profiles. These measurements inform critical policy decisions, are integrated into sustainability efforts for fishers, scientists, and other relevant stakeholders, and can improve climate resiliency while protecting the health, well-being, and livelihoods of coastal communities and participants in the blue economy.
As described in a recent publication, the Fishing Vessel Ocean Observing Network (FVON) is reimagining the global data collection paradigm of coastal and shelf oceans by partnering with fishers and regional observation networks around the world. With more than four million fishing vessels worldwide, fishers cover much of the data-sparse nearshore ocean environments, vitally important regions of the ocean. By outfitting sensors onto vessels and on fishing gear, programs from New Zealand to Japan to New England, including researchers at WHOI, demonstrate that fishers can participate actively in the ongoing data revolution and eliminate critical oceanic data gaps without changing their standard fishing activities. Exponentially increasing the scale of data collection through fishing vessel and gear-based observations in nearshore marine environments has and will continue to democratize ocean observation, improve weather forecasting and ocean monitoring, and promote sustainable fishing while safeguarding lives and livelihoods. Already a proven concept regionally, FVON, alongside fishers and regional observation networks, will expand fishing-based observation to a global initiative.
Authors
Cooper Van Vranken (Ocean Data Network)
Julie Jakoboski (MetOcean Solutions, New Zealand)
John W. Carroll (Ocean Data Network)
Christopher Cusack (Environmental Defense Fund)
Patrick Gorringe (Swedish Meteorological and Hydrological Institute)
Naoki Hirose (Kyushu University, Japan)
James Manning (NOAA Northeast Fisheries Science Center (retired))
Michela Martinelli (National Research Council−Institute of Marine Biological Resources and Biotechnologies, Italy)
Pierluigi Penna (National Research Council−Institute of Marine Biological Resources and Biotechnologies, Italy)
Mathew Pickering (Environmental Defense Fund)
A. Miguel Piecho-Santos (Portuguese Institute for Sea and Atmosphere)
Moninya Roughan (University of New South Wales, Australia)
João de Souza (MetOcean Solutions, New Zealand)
Hassan Moustahfid (NOAA Integrated Ocean Observing System (IOOS))