Rapid environmental changes in the Arctic will potentially alter the atmospheric emissions of heat-trapping greenhouse gases such as methane (CH4) and carbon dioxide (CO2). A recent study on the Canadian Arctic published in Geophysical Research Letters reveals that spring meltwater delivery drives episodic outgassing events along the lake-river-bay continuum. This spring runoff period is not well-represented in prior studies, which, due to ease of sampling access, have focused more on summertime low-ice conditions. Study authors established a community-based monitoring program in Cambridge Bay and an adjacent inflowing river system in Nunavut, Canada from 2017-2018. These time-series data revealed that at the onset of the melt season river water contains methane concentrations up to 2000 times higher than observed in the bay from late summer through early spring (Figure 1 panel a). In addition, the authors deployed a novel robotic chemical sensing kayak (the ChemYak) in the Bay for five days in 2018 to densely sample water CH4 and CO2 levels in space and time during the spring thaw (Figure 1 panel b). The ChemYak observations revealed that river water containing elevated levels of both of these greenhouse gases flowed into the bay and outgassed to the atmosphere over a period of 5 days! The authors estimate that river inflow during the short melt season drives >95% of all annual methane emissions from the bay. These results demonstrate the need for seasonally-resolved sampling to accurately quantify greenhouse gas emissions from polar systems.
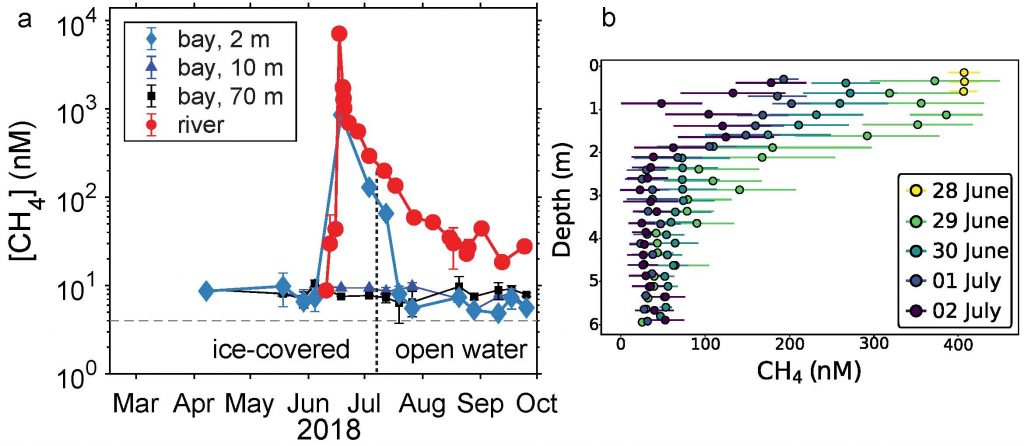
Figure 1: Panel a) Measurements of methane concentration in Cambridge Bay and an adjacent river showed strong seasonality; elevated concentrations were associated with river inflow at the start of the freshet. Panel b) Observations with the ChemYak robotic surface vehicle in Cambridge Bay revealed that excess methane was rapidly ventilated to the atmosphere following ice melt in the bay.
Authors
Cara Manning (University of British Columbia)
Victoria Preston (Woods Hole Oceanographic Institution and Massachusetts Institute of Technology)
Samantha Jones (University of Calgary)
Anna Michel (Woods Hole Oceanographic Institution)
David Nicholson (Woods Hole Oceanographic Institution)
Patrick Duke (University of Calgary and University of Victoria)
Mohamed Ahmed (University of Calgary)
Kevin Manganini (Woods Hole Oceanographic Institution)
Brent Else (University of Calgary)
Philippe Tortell (University of British Columbia)