Gelatinous zooplankton comprise a widespread group of animals that are increasingly recognized as important components of pelagic ecosystems. Historically understudied, we have little knowledge of how much key taxa contribute to carbon fluxes. Likewise, there’s a critical knowledge gap of the impact of ocean change on these taxa.
Appendicularia are the most abundant gelatinous zooplankton in the world oceans. Their population dynamics display typical boom-and-bust characteristics, i.e. high grazing rates in combination with a short generation time and life cycle, results in intense blooms. The most prominent feature of appendicularians is their mucous feeding-structure (“house”), which is produced and discarded several times per day. These sinking houses can contribute substantially to carbon export.
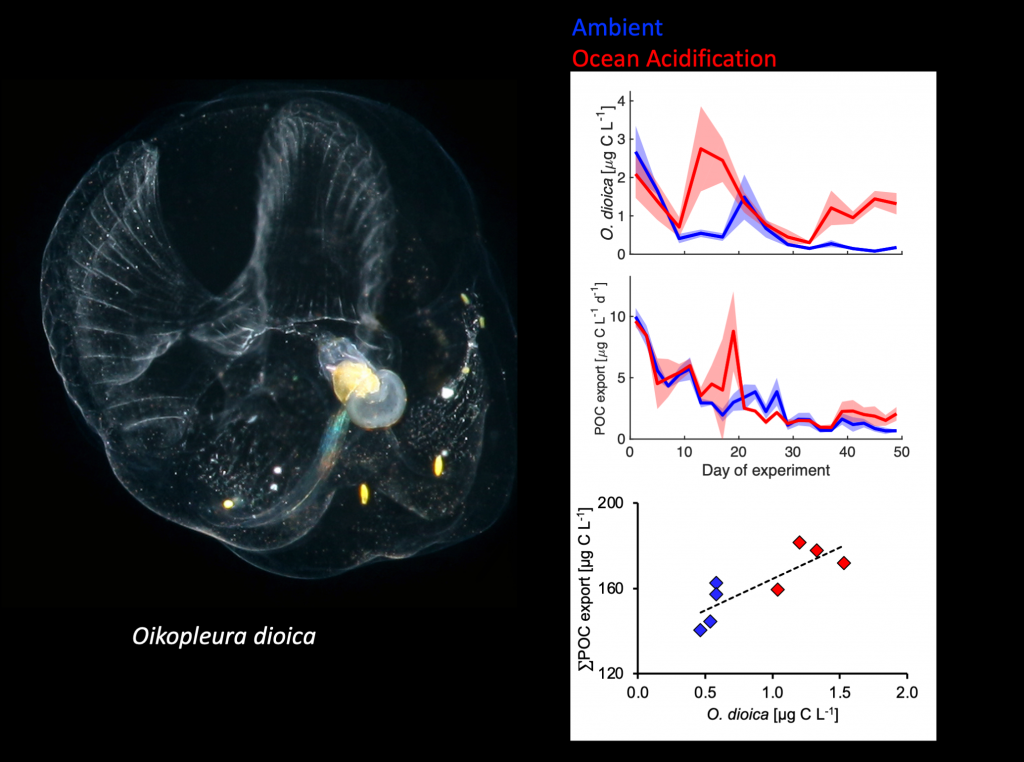
Figure 1: Influence of ocean acidification on the Appendicularia Oikopleura dioica and carbon export. Appendicularian populations display typical boom-and-bust characteristics, resulting in intense blooms. The sinking of appendicularians’ discarded mucous feeding-structure several times per day can contribute substantially to carbon export. Low pH conditions (as expected for future ocean acidification extreme events) enhanced its population growth and contribution to carbon fluxes shown above (red lines/diamonds) vs ambient (blue lines/diamonds).
(Figure sources: Picture by Jean-Marie Bouquet, data plots from Taucher et al. (2024): The appendicularian Oikopleura dioica can enhance carbon export in a high CO2 ocean. Global Change Biology, doi:10.1111/gcb.17020)
A recent study in Global Change Biology quantified how much appendicularia can contribute to carbon export via the biological pump, and how this carbon flux could markedly increase under future ocean acidification and associated extreme pH events.
The findings are based on a large-volume in situ experimental approach that allowed observing natural plankton populations and carbon export under close-to-natural conditions for almost two months. Thereby, O. dioica population dynamics could be directly linked to sediment trap data to quantify the influence of this key species on carbon fluxes at unprecedented detail. During the appendicularia bloom up to 39% of total carbon export was attributed to them.
The most striking finding was that high CO2 conditions elevated carbon export by appendicularia increased by roughly 50%. Appendicularians physiologically benefit from low pH conditions, giving them a competitive advantage over other zooplankton, allowing them to contribute to a disproportionally large role in carbon export from the ecosystem.
Authors
Jan Taucher (GEOMAR)
Anna Katharina Lechtenbörger (GEOMAR)
Jean-Marie Bouquet (University of Bergen)
Carsten Spisla (GEOMAR)
Tim Boxhammer (GEOMAR)
Fabrizio Minutolo (GEOMAR)
Lennart Thomas Bach (University of Tasmania)
Kai T. Lohbeck (University of Konstanz)
Michael Sswat (GEOMAR)
Isabel Dörner (GEOMAR)
Stefanie M. H. Ismar-Rebitz (GEOMAR)
Eric M. Thompson (University of Bergen)
Ulf Riebesell (GEOMAR)