Marine fishes and filter-feeding gelatinous zooplankton such as salps and pyrosomes generate detritus in the form of poop and dead carcasses, which sink ~10 times faster than other oceanic detritus. This detritus is hypothesized to have a disproportionally large impact on the marine biological pump as it sequesters carbon and nutrients deeper in the water column. Until now, global models had not considered these fluxes, thus, their impacts on ocean biogeochemical cycles were not well understood.
A recent study in Geophysical Research Letters investigated the sensitivity of deep ocean carbon, oxygen, and nutrient cycles to fast-sinking detritus from filter-feeding gelatinous zooplankton (pelagic tunicates) and fishes, using a modified version of the NOAA-GFDL ocean biogeochemical model COBALT (“GZ-COBALT”). We found the fast-sinking detritus decreased surface productivity and export, while increasing transfer efficiency and sequestration at depth. Ocean oxygen minimum zones (OMZs) also decreased in size: fast-sinking detritus triggered less remineralization, particularly in the mid-depths, resulting in less oxygen consumption and a reduced expansion of OMZs.
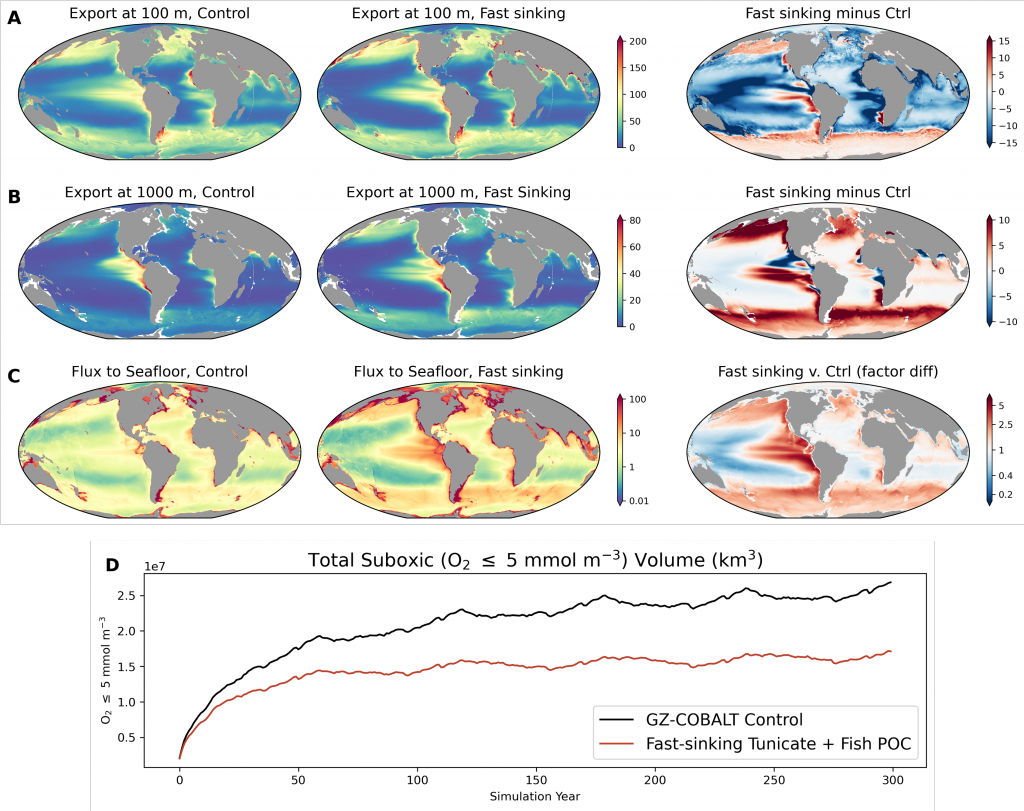
Figure caption: Flux of detrital carbon at various depths (A, B, C), shows that incorporating fast-sinking detritus counter-intuitively decreases carbon export from the surface while increasing sequestration at depth. Particulate organic carbon (POC) export flux at (A) 100 m, (B) 1,000 m and (C) seafloor (mgC/m2/d), shows (left) the control simulation with no fast-sinking detritus, (center) the experiment with fast-sinking fish and gelatinous zooplankton detritus, and (right) the differences between the control and fast-sinking detritus simulation. (D) Total ocean volume over the 300-year simulation at the suboxic (O2 ≤ 5 mmol/m3) level, shows the simulation with fast-sinking particulate organic carbon (red) had lower suboxia than the control (black). Large hypoxic and suboxic zones are a common model bias; these results suggest that fast-sinking detritus may be one biogeochemical mechanism to reduce the expansion of these low oxygen zones.
Past observations have shown that fast-sinking, highly reactive detritus reaching the seafloor can fuel significant benthic consumption and respiration. On a global scale, we suggest that the increased fluxes to the seafloor in the model can be supported by observational constraints of seafloor oxygen consumption, suggesting that these processes could be realistically incorporated into future generations of Earth System Models.
Authors
Jessica Y. Luo (NOAA GFDL)
Charles A. Stock (NOAA GFDL)
John P. Dunne (NOAA GFDL)
Grace K. Saba (Rutgers University)
Lauren Cook (Rutgers University)