Microscopic plankton in the surface ocean make planet Earth habitable by generating oxygen and forming the basis of marine food webs, yielding harvestable protein. For over 100 years, oceanographers have tried to ascertain the physical, chemical, and biological processes governing phytoplankton blooms. Zooplankton grazing of phytoplankton is the single largest loss process for primary production, but empirical grazing data are sparse and thus poorly constrained in modeling frameworks, including assessments of global elemental cycles, cross-ecosystem comparisons, and predictive efforts anticipating future ocean ecosystem function. As sunlight decays exponentially with depth, upper-ocean mixing creates dynamic light environments with predictable effects on phytoplankton growth but unknown consequences for grazing.
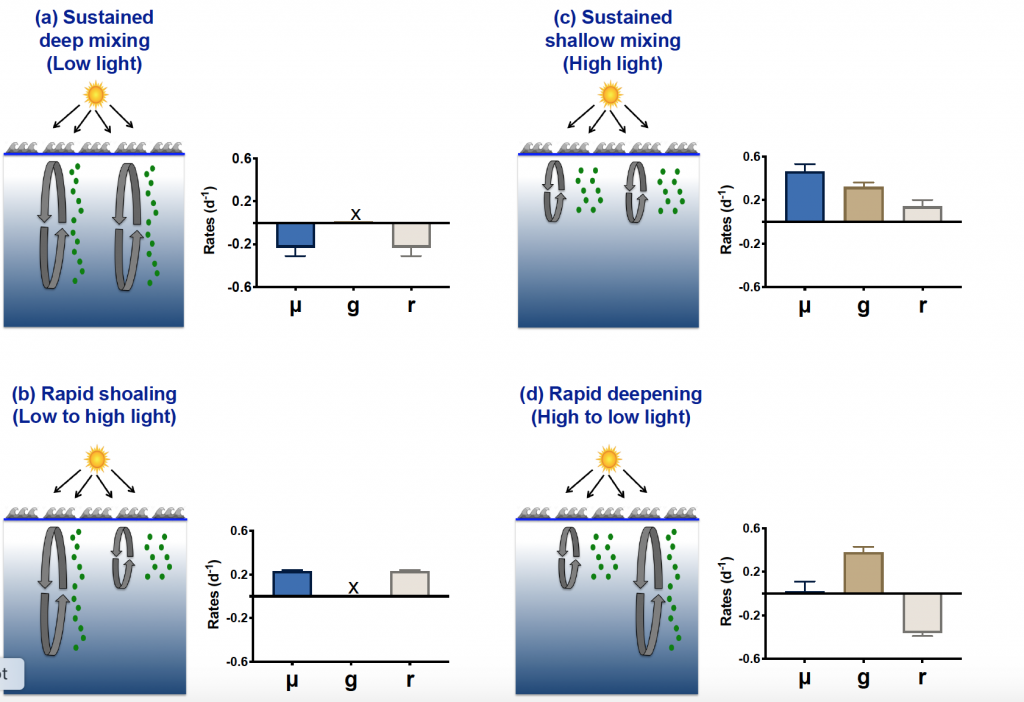
Figure caption: Rates (d−1) of phytoplankton growth (μ), grazing mortality (g), and biomass accumulation (r) under four mixed layer scenarios simulated using light as a proxy of (a) sustained deep mixing, (b) rapid shoaling, (c) sustained shallow mixing, and (d) rapid mixed layer deepening. Error bars represent one standard deviation of the mean of duplicate experiments. Grazing was measured but not detected in the sustained deep mixing and rapid shoaling conditions, denoted with x.
Using data from a spring cruise in the North Atlantic, authors of a recent study published in Limnology & Oceanography compared the influences of microzooplankton predation and fluctuations in light availability—representative of a mixing water column—on phytoplankton standing stock. Data from at-sea incubations and light manipulation experiments provide evidence that phytoplankton’s instantaneous and zooplankton’s delayed responses to light fluctuations are key modulators of the balance between phytoplankton growth and grazing rates (Figure 1). These results suggest that light is a potential, remotely retrievable predictor of when and where in the ocean zooplankton grazing may represent an important loss term of phytoplankton production. If broadly verified, this approach could be used to systematically assess sparsely measured grazing across spatial and temporal gradients in representative regions of the ocean. Such data will be essential for enhancing our predictive capacity of ocean food web function, global biogeochemical cycles and the many derived processes, including fisheries production and the flow of carbon through the oceans.
Authors:
Françoise Morison (University of Rhode Island)
Gayantonia Franzè (University of Rhode Island, currently Institute of Marine Research, Norway)
Elizabeth Harvey (University of Georgia, currently University of New Hampshire)
Susanne Menden-Deuer (University of Rhode Island)