In the Southern Ocean, coccolithophores are thought to account for a major fraction of marine carbonate production and export to the deep sea. Despite their importance in the ocean carbon cycle, we lack fundamental information about Southern Ocean coccolithophore abundance, species composition, and contribution to carbonate export.
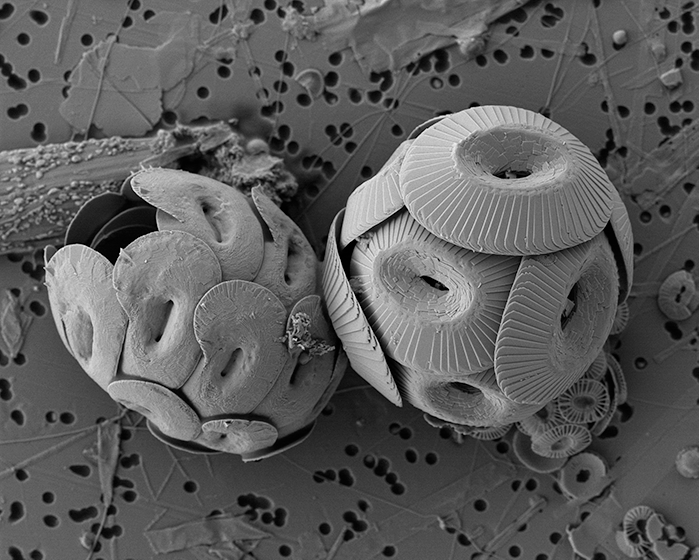
Figure caption: Heliscosphaera carteri (left), Coccolithus pelagicus (right) and Emiliania huxleyi (bottom right, partially behind C. pelagicus) coccospheres retrieved from the subantarctic waters south of Tasmania. Image Ruth Eriksen, courtesy AAD EMU.
A recent study in Biogeosciences has generated annual observations of coccolithophore species composition and contribution to calcium carbonate fluxes at two sites that are representative of a large portion of the Subantarctic zone. Coccolithophores account for roughly half of the annual calcium carbonate exported to the deep sea. Notably, it is not the most abundant species (Emiliania huxleyi), but rather the less abundant and larger species (e.g. Calcidiscus leptoporus, Helicosphaera carteri and Coccolithus pelagicus) that make the greatest contribution to carbonate export to the deep sea. Since these larger species exhibit substantially different ecological traits from the opportunistic E. huxleyi, predictions of future response of Southern Ocean coccolithophore communities should not be based on the physiological results from experiments with E. huxleyi. Rather, new physiological response experiments of those less abundant, larger coccolithophore species are urgently needed to constrain responses of these important carbonate exporters to environmental change in the Southern Ocean. This study underscores the importance of phytoplankton ecological traits on the regulation of the marine carbon cycle and emphasizes the need for more species-specific studies to improve predictions of marine ecosystem response to ongoing climate change.
Authors
Andrés S. Rigual Hernández (Universidad de Salamanca)
Thomas W. Trull (CSIRO and ACE CRC)
Scott D. Nodder (NIWA)
José A. Flores (Universidad de Salamanca)
Helen Bostock (University of Queensland,)
Fátima Abrantes (Portuguese Institute for Sea and Atmosphere and CCMAR)
Ruth S. Eriksen (CSIRO and IMAS)
Francisco J. Sierro (Universidad de Salamanca)
Diana M. Davies (CSIRO and ACE CRC)
Anne-Marie Ballegeer (Universidad de Salamanca)
Miguel A. Fuertes (Universidad de Salamanca)
Lisa C. Northcote (NIWA)