Zooplankton-mediated carbon export is an important, but variable and relatively unconstrained part of the biological carbon pump—the processes that fix atmospheric carbon dioxide in organic material and transport it from the upper sunlit ocean to depth. Changes in the biological pump impact the climate system, but are challenging to quantify because such analyses require spatially and temporally explicit information about biological, chemical, and physical properties of the ocean, where empirical observations are in short supply.
A recent study in Nature, Ecology and Evolution focused on copepods in the northern half of the North Atlantic Ocean, where the Continuous Plankton Recorder (CPR) time series program has documented surface plankton abundance and taxonomic composition for nearly six decades. Copepods transport carbon passively by producing sinking fecal pellets while feeding near the sea surface, and actively via daily and seasonal migrations to deeper waters where carbon is released through respiration, defecation, and mortality. Using allometry, metabolic theory, and an optimal behavior model, the authors examined patterns of passive and active carbon transport from 1960 to 2014 and sensitivity of carbon export to different model inputs.
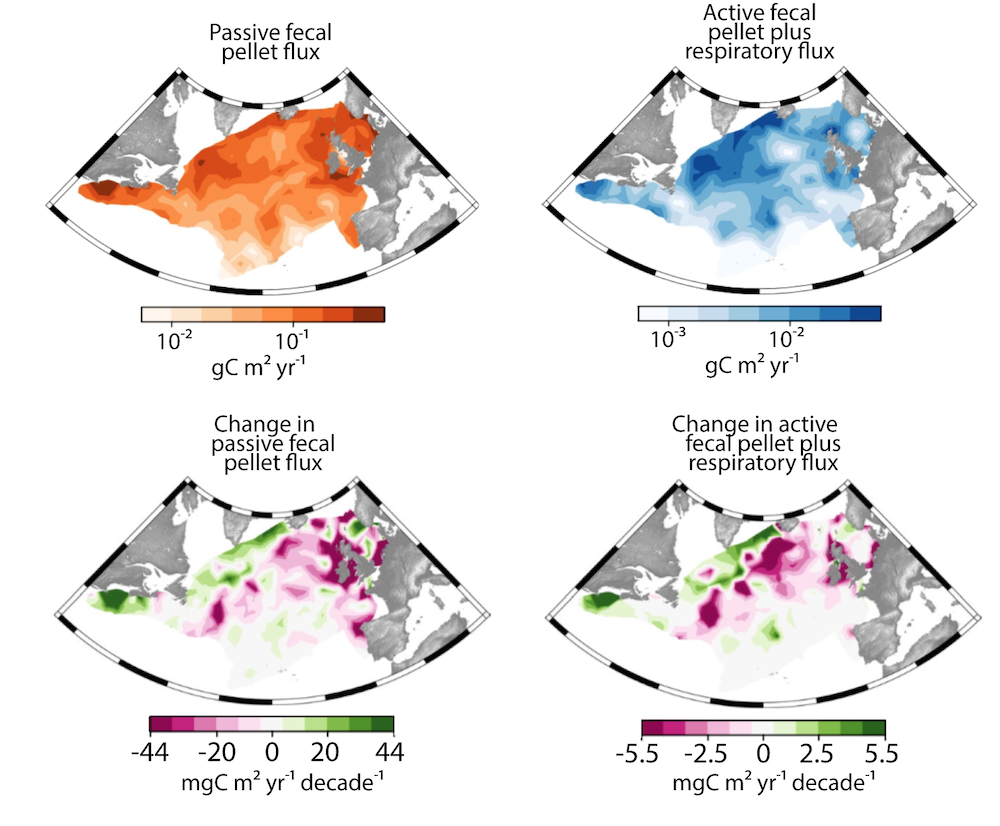
Figure caption: Spatial distribution and change, from 1960 to 2014, of modeled copepod-mediated carbon flux: top left – mean passive carbon flux (sinking fecal pellets), bottom left – change in passive carbon flux, top right – mean active carbon flux (respiration plus fecal pellets produced during diel vertical migration), bottom right – change in active carbon flux.
The authors observed that from southern Iceland to the Gulf of Maine, copepod-mediated carbon transport has increased over the last six decades, with the highest rates around 30 mgC m-2 y-1 each decade for passive flux, and 4 mgC m-2 y-1 each decade for active flux. Meanwhile, it has decreased across much of the more temperate central northern North Atlantic with highest rates around 69 mgC m-2 y-1 each decade for passive flux and 8 mgC m-2 y-1 each decade for active flux. This pattern is largely driven by changes in copepod population distributions and community structure, specifically the distributions of large and abundant species (e.g. Calanus spp.). These results suggest that shifts in species distributions driven by a changing global climate are already impacting ecosystem function across the northern North Atlantic Ocean. These shifts are not latitudinally uniform, thus highlighting the complexity of marine ecosystems. This study demonstrates the importance of these sustained plankton measurements and how plankton-mediated carbon fluxes can be mechanistically implemented in next-generation biogeochemical models.
Authors:
Philipp Brun (Technical University of Denmark and Swiss Federal Research Institute)
Karen Stamieszkin (University of Maine, Bigelow Lab and Virginia Institute of Marine Science)
Andre W. Visser (Technical University of Denmark)
Priscilla Licandro (Sir Alister Hardy Foundation for Ocean Science, Plymouth Marine Laboratory, and Stazione Zoologica Anton Dohrn, Italy)
Mark R. Payne (Technical University of Denmark)
Thomas Kiørboe (Technical University of Denmark)
Also see OCB2019 plenary session: The effect of size on ocean processes (allometry) and implications for export (Thursday, June 27, 2019)